ENTER THE CARNIVORES
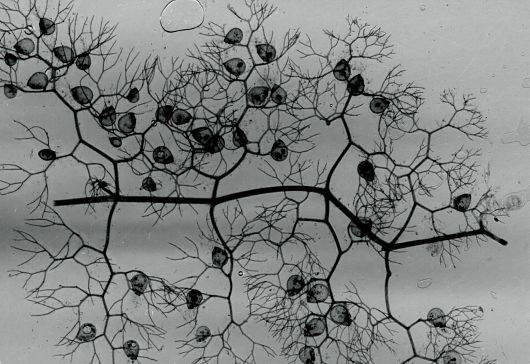
The humble Bladderwort, Utricularis. Photo: Ulrike Muller
By Caroline Wood
"Every time I look at these plants, I find something new and fascinating”, so said Charles Darwin of carnivorous plants. Since his time, these captivating species have inspired countless botanists and plant scientists.
Despite this, there hadn’t been a dedicated session on carnivorous plants at an SEB meeting for nearly 40 years, the last being in 1980. “Carnivorous plants – Physiology, ecology, and evolution” at the 2017 SEB Annual Meeting put this right. Touching on all manner of fields from ecology, biomechanics, biomaterials and developmental biology, there was plenty of cutting-edge research for the delegates to get their teeth into.
MARVELLOUS MOVERS
The most iconic carnivorous plant has to be the Venus Flytrap, Dionaea muscipula, which Darwin called “the most wonderful plant in the world”. Even today, the swift closing action of the lobed trap is mesmerising: “I was fascinated by the Venus Flytrap as a child and have been stuck to carnivorous plants ever since”, said Simon Poppinga, of the Plant Biomechanics Group at University of Freiburg, Germany. Indeed, Simon’s passion for carnivorous plants led him to research how these organisms can move so quickly without muscles. It’s now known that the Venus Flytrap employs a snap-buckling instability, as Simon describes: “The lobes of the trap are doubly curved and can rapidly flip from a convex to a concave configuration in as little as 100 milliseconds”.
Yet the Venus Flytrap is woefully slow compared to its aquatic sister, Aldrovanda vesiculosa, otherwise known as the Waterwheel Plant, whose underwater snap traps can close in only 20–50 milliseconds. Despite its morphological similarity to Dionaea, Simon and PhD student Anna Westermeier (both from the Plant Biomechanics Group Freiburg, Germany) have found that Aldrovanda uses a completely different mechanism to close its traps. Using high-speed cameras mounted on stereo microscopes, Anna analysed the movement of traps placed between two electrodes that were triggered to close using electrical impulses. This demonstrated that the process relies on movement amplification, where a small deformation of the central midrib is magnified into the lobes. It is presumed that the initial deformation is driven by a loss of turgor pressure in the cells adjacent to the midrib, similar to other hydraulically-driven plant movements, such as the closing of guard cells in stomata. But the sheer speed of closure suggested that this wasn’t the complete answer. As Anna explained: “Trap closure in Aldrovanda is almost as fast as plant movements that rely on elastic instabilities, rather than purely hydraulic forces. Therefore, the question arose if Aldrovanda also incorporates pre-stresses to boost its speed.” To investigate this further, Anna had to team up with colleagues that had expertise in computer simulations. “We used a method called Finite Element Analysis, where the trap is reduced to the minimum elements necessary for the movement”, she said. “We can then manipulate certain components and recalculate the stresses and compressions acting on the trap. It’s very powerful as you can perform manipulations you could never do on a real trap”. Using this approach, they developed a physical model where the plant actively incorporates pre-stresses into the trap before it is triggered.“You can think of the midrib like an inverted bow”, explained Simon. “Normally, a bow is pre-stressed into a curved state and then the elastic energy is released when we fire it. In Aldrovanda, it is the other way around: the trap is kept open using turgor pressure and when this is released, the midrib relaxes into a curved state and forces the lobes to move towards each other, closing the trap”.
Indeed, such is the elegance of Aldrovanda’s trap mechanism that its kinetics have inspired a new adaptive shading device: the Flectofold. This biomimetic façade shading system was developed and built in the framework of the Collaborative Research Centre 1411 with engineers and architects from the University of Stuttgart, Germany. The stiff flaps are connected to the midrib along curved fold lines. The closing movement is driven by a pneumatic cushion which induces bending in the midrib that causes an amplified folding movement of the shading flaps2.
GETTING SUCKED IN
However, when it comes to trap speed, Aldrovanda would only get the silver medal. The fastest known carnivorous plant is the humble Bladderwort, Utricularia, an aquatic plant that captures zooplankton in tiny suction traps. “They are so easy to overlook – they look just like green snot”, said fluid mechanist Ulrike Muller (California State University Fresno, United States). Yet her work has found that the tiny traps pack a suction force mighty enough to break the cohesive forces of water. “For small organisms, the main property of water that affects movement is its viscosity, caused by the attraction between water molecules”, explained Ulrike. This attraction causes water molecules to form a layer around objects that move through it, generating viscous forces. Because Bladderwort traps are only a few millimetres in size, they ought to be subject to viscous forces; as Ulrike said “for this to be otherwise would be a special achievement”. To make the suction flow visible, she added small tracer particles to the water surrounding Utricularia and illuminated them with a narrow laser beam. The traps were then filmed using high-speed cameras operating at 50,000 frames per second. “From this, we could see that the traps generate flow patterns that look exactly the same as the flow predicted by inviscid flow models”, said Ulrike. “Basically, the speed of the trap overcomes the viscous force of water, meaning that the water molecules simply don’t have time to stick to one another”.
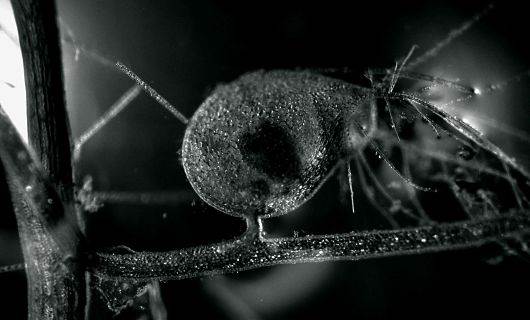
The trap of Bladderwort, Utricularia - The fasterest carnivorious plant on record! Photo; Ulrike Muller
This means that compared to animals that feed using suction forces, Utricularia are in a league of their own. “Bladderwort traps are the same size as fish larvae, which also feed using suction forces”, explained Ulrike. “But whilst the Bladderwort reaches peak pressure in less than a millisecond, it takes fish larvae up to 10 milliseconds. To pardon the pun – fish suck at sucking!” But how the Bladderwort achieves this is currently a mystery. “Our best hypothesis at the moment is elastic energy storage”, said Ulrike. “But we have no proof for this, so we are now investigating the structures and materials in the trap to see if there are any candidates for storing elastic energy”. Like Anna, Ulrike plans to use Finite Element Analysis on computer models of the Utricularia trap to manipulate the stiffness and thickness of different components.
SLIPPERY CUSTOMERS
Speed isn’t the only mechanism carnivorous plants use: in many species, the traps do not move at all and passively wait for their victims to stumble into them. Perhaps the most wellknown are the Pitcher Plants of the genus Nepenthes, whose traps consist of a fluidfilled cavity containing digestive enzymes. Nectar-secreting glands entice insects to the rim (known as the peristome), from which many fall to their doom. Understanding how the peristome is so slippery is the focus of research being conducted by Ulrike Bauer (University of Bristol, UK). “The surface of the peristome is unusually wettable”, she said. “Instead of forming beads, water droplets that fall on the surface spread out as an even layer”. Under a Scanning Electron Microscope, it can be seen that the peristome is made up of regular microscopic ridges of smooth overlapping epidermal cells, separated by grooves3. Water spreads along these ridges through a natural capillary action, forming a homogenous thin film. The microstructures of the peristome cause the solid and liquid surface energies to be closely matched, holding the overlying water film in a highly stable state. By placing Weaver Ants on the peristome that were connected by a thread to a tiny strain gauge force transducer, Ulrike’s colleague Holger Bohn confirmed that the combination of surface topography and overlying liquid film prevents the claws and adhesive pads of the ants from getting a grip, similar to a car aquaplaning.
These adaptations are inspiring new biomaterials in the form of Slippery Liquid- Infused Porous Devices (SLIPs). Traditionally, water-repelling surfaces use microstructures to repel liquids directly, however SLIPs mimic Nepenthes by using a microporous substance to lock in an intermediary film of liquid which itself becomes the repellent surface. It is hoped that this could be used in applications as varied as medicine, fluid transportation and self cleaning materials for extreme environments4.
FIGHTING BACK
With such sophisticated traps, it’s easy to feel sorry for the insects and other prey species that coexist with carnivorous plants, but it seems that they have their own ways of fighting back. Indeed, during the session, Sebastian Kruppert (Ruhr-Universität Bochum, Germany) presented the first known case of a plant driving an inducible defence in an animal species. “We already knew that the presence of animal predators can induce Daphnia water fleas to change their morphology and increase their carapace’s stiffness up to two times”, he said. A chance meeting with Simon Poppinga during the Biomechanics Session at the 2015 SEB Annual Meeting in Prague inspired him to investigate whether predation by Utricularia could have the same effect. However, their initial results were surprising, as it appeared that Ceriodaphnia dubia that were raised in the presence of Utricularia were actually smaller than the controls. “This was curious because Utricularia can only catch organisms that fit within the trap, so prey species should aim to be bigger not smaller”, Sebastian said. However, it transpired that measuring Daphnia the traditional way – diagonally from the eye to the tail spine – was giving misleading data. “We then used a 3D-modelling system with confocal laser scanning microscopy developed by my colleague Martin Horstmann”, continued Sebastian. “This projects a point cloud onto the image which maps the surface”. Using this method, it became clear that Ceriodaphnia raised with Bladderworts were becoming broader with a larger cross-sectional area. In addition, they developed lateral fornices (spine-like structures) on the head, further increasing the lateral width. “These changes would make it more difficult for the Ceriodaphnia to physically fit in the trap”, Sebastian explained. This was confirmed using predation trials: morphologically adapted Ceriodaphnia were significantly less susceptible to Utricularia predation than non-adapted controls raised in predator-free conditions.
How Daphnia detect the presence of Utricularia is currently a mystery. “Possibly, Utricularia give off a chemical signal because we know that daphniids respond to chemicals released by animal predators. It needs more research, and my colleagues are currently working on this pathway”, said Sebastian. “We are also currently investigating whether the presence of Utricularia induces behavioural changes in Daphnia, as these would be quicker to use than morphological changes, which take a couple of days”.
MORE OF A VEGETARIAN AFTER ALL?
Despite this apparent arms race between Bladderworts and their Daphnia prey, carnivory may actually only play a minor role in nutrient acquisition for these plants. “Studies suggest that the rate of invertebrate prey capture is not enough to sustain the growth of Utricularia at many locations” said Dagmara Sirova (University of South Bohemia, Czech Republic). Instead, Bladderworts may rely on the complex and interconnected ecosystem of algae, bacteria and protozoan species contained within their traps. But analysing this system is no easy task, as Dagmara explained: “As the traps are so small, we had to develop a suction system with a thin glass capillary tube attached to a peristaltic pump. It took hundreds of trap samples to get 1 ml of fluid.” Using a variety of approaches, including epifluorescence microscopy, stable isotope labelling, metagenomics and metatranscriptomics, the results suggested that over 4,000 species can be found in the tiny traps, a diversity on a par with the soil rhizosphere. Despite this complexity, computer modelling and clustering analysis has shed light on the key players within this soup. In particular, the trap’s communities show some similarities to the guts of mammalian ruminants, including an abundance of various bacterial species able to degrade complex plant polymers. This suggests that most of Utricularia’s nutrition may actually be algal in origin. “Various algal species grow on the submerged leaves of Utricularia, and disturbances can cause them to fall into the trap”, explained Dagmara. “However, the nutrients released from this captured organic matter don’t benefit the plant directly as they are first used by the trap-colonizing bacteria”. It seems that the recently described ciliate Tetrahymena utriculariae is a grazer that is key for completing the cycle, as it acts as a nutrient regenerating machine. “Using fluorescently labelled bacteria, we found that Tetrahymena utriculariae can overturn the entire standing stock of bacteria five times a day”, said Dagmara. “It’s a very efficient but sloppy feeder, which releases nutrients in mineral form, readily available for plant uptake”. Further evidence for the importance of these microbial communities comes from the fact that young traps (which are initially sterile), exude up to 25% of their photosynthates into the trap lumen to attract bacteria. According to Dagmara, this makes sense when one considers the nutrient-poor areas where Utricularia thrives: “In these places, metazoan prey is often scarce but the supply of algae is continuous and abundant. As such, Utricularia-microbe systems should be considered as synchronized, mutually dependent biological and ecological units”.
A PERFECT LITTLE SYSTEM TO ASK BIG QUESTIONS
Similar to Utricularia, Pitcher Plants also contain multi-trophic communities of microorganisms which make “good systems for asking the big questions that have preoccupied ecologists for hundreds of years”, according to Aaron Ellison (Harvard University, United States). “We can think of Pitcher Plants as small experimental lakes that can be manipulated. You can have several hundred Pitcher Plants in a single metre squared, each one being a single replicate”, he said. “For an experimental ecologist interested in aquatic systems, it’s a very attractive system. Pitcher Plants also have the advantage that their food webs can be built from the bottom up. This means we can ask what factors control the structure of a food web: is it how much space there is, the amount of resources or key stone predators?” More recently, Aaron has used the carnivorous Northern Pitcher Plant, Sarracenia purpurea to examine how healthy lakes change to oxygenpoor, eutrophic systems. “At the moment, it takes 40 years advanced warning to prevent a state change in a lake, but Pitcher Plants allow us to investigate these dynamics on a much quicker time-scale”, he said. In unfed plants, the concentration of dissolved oxygen shows a regular diurnal cycle with levels high during the day when photosynthesis occurs, and lower at night when the plants respire. As soon as prey is introduced, these regular cycles are disrupted due to the decomposing activity of the microorganisms within the trap. By comparing plants with different rates of prey addition, Aaron demonstrated that the rate of prey decomposition and the biological oxygen demand were the primary drivers that triggered the transition to an anaerobic state5. “We found that this transition could be induced in as little as 70 hours”, said Aaron. “Our hope now is to develop biomarkers for tipping points so that we can anticipate these changes more rapidly in lake systems”.
LOOKING TO THE FUTURE…
A final use for carnivorous plants that should not be overlooked is their capacity to captivate audiences across the globe and inspire the public and even the next generation of plant scientists. “Carnivorous plants are a good entry point to get the public talking about science”, said Aaron, who helped develop a freely-available downloadable teaching resource on carnivorous plants with the Linnean Society6. Many rare carnivorous plants are monitored as part of citizen science programmes, where members of the public record sightings to online databases. A new species of Drosera sundew was even discovered on Facebook in 2015, spotted by PhD student Paulo Gonella in a photo of Brazilian plants posted on a friend’s newsfeed7. Given that so many carnivorous species are threatened by habitat loss, it is vital that more people are inspired to help protect them. As SEB researchers have shown, they deserve to be seen as a ‘Cabinet of Curiosities’ rather than the ‘Little Shop of Horrors’.
Hungry for more? The last SEB Carnivorous Plants session led to the publication of ‘The Carnivorous Plants’, by Juniper, Robins and Joel (Academic Press, 1988). Fittingly, many of the speakers for the 2017 session have contributed to the forthcoming book ‘ Carnivorous Plants: Physiology, ecology, and evolution’, edited by Aaron Ellison and Lubomír Adamec. It is scheduled to be published in January 2018 by Oxford University Press.
1. http://www.trr141.de/
2. Poppinga, S.; Körner, A.; Sachse, R.; Born, L.; Westermeier, A.S.; Hesse, L.; Knippers, J.; Bischoff, M.; Gresser, G.; Speck, T. Compliant mechanisms in plants and architecture. In: Knippers, J.; Nickel, K.G.; Speck, T. (eds) Biomimetic Research for Architecture and Building Construction: Biological Design and Integrative Structures. Springer book series “Biologically- Inspired Systems”, vol. 9. Springer International Publishing AG, Switzerland (2016), pp 169–19.
3. Bohn, Holger F., and Walter Federle. “Insect aquaplaning: Nepenthes pitcher plants capture prey with the peristome, a fully wettable waterlubricated anisotropic surface.” Proceedings of the National Academy of Sciences of the United States of America 101.39 (2004): 14138–14143.
4. Wong, Tak-Sing, et al. “Bioinspired selfrepairing slippery surfaces with pressure-stable omniphobicity.” Nature 477.7365 (2011): 443.
5. Sirota, Jennie, et al. “Organic-matter loading determines regime shifts and alternative states in an aquatic ecosystem.” Proceedings of the National Academy of Sciences 110.19 (2013): 7742–7747.
6.https://www.linnean.org/education-resources/ secondary-resources/darwin-inspired-learning/ murderous-plants
7.http://www.telegraph.co.uk/news/worldnews/ southamerica/brazil/11762745/New-plant-speciesdiscovered- on-Facebook.html